The great potential that nanotechnology offers enables the progress of new devices in the nanodiagnostic field, mainly in nanobiosensors.
Their properties are characterised by the nanoscale at which they are produced. Unlike biosensors , which we studied in the post: "Introduction to biosensors", where their sensory receptors are distanced by hundreds of micrometers, here they are distanced by a few nanometers.
Their properties are characterised by the nanoscale at which they are produced. Unlike biosensors , which we studied in the post: "Introduction to biosensors", where their sensory receptors are distanced by hundreds of micrometers, here they are distanced by a few nanometers.
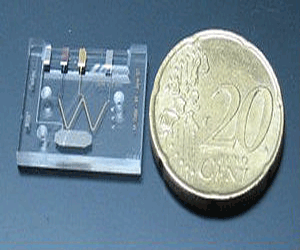
Thus, even sensors constituted by single molecules bound to their surface have been designed. Consequently the process of diagnosis is performed with a much smaller size device, improving portability and the potential for use anywhere.
Also, only extremely small sample quantities (micro or nanolitres) are required to carry out the analysis, so the sample extraction methods are less traumatic and invasive for patients.
Also, only extremely small sample quantities (micro or nanolitres) are required to carry out the analysis, so the sample extraction methods are less traumatic and invasive for patients.
These instruments also facilitate the use of samples without fluorescent or radioactive markers, which are used in biological and clinical analysis. This property is essential, since it has been proven that when a sample is not marked or altered before examining, the detection sensitivity significantly increases.
Within nanobiosensors we can distinguish the following categories:
Within nanobiosensors we can distinguish the following categories:
Nanophotonic biosensors
This class of nanobiosensors, also known as evanescent wave biosensors, is based on how the light is transmitted by multiple internal reflections through the optical waveguide, which is a component of the biosensor.
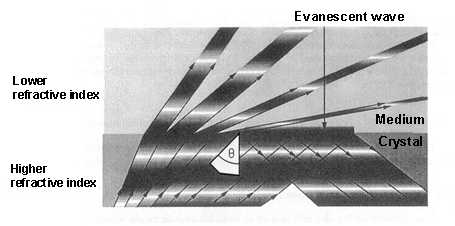
With these devices, only a few microliters of sample are necessary to determine protein concentrations or the variations of just one DNA base.
Also, it is possible to evaluate the metabolic state of just a single cell because some of these nanobiosensors have sharp fibre optic (30-50 nm) filaments, which can be introduced into cells through the plasma membrane without harming the cell.
This method facilitates the study of in vivo cell functions (apoptosis[2], cell division, biological nanomachines…) and it can detect pathological changes in a single cell.
This method facilitates the study of in vivo cell functions (apoptosis[2], cell division, biological nanomachines…) and it can detect pathological changes in a single cell.
Nanoplasmonic biosensors
In the Surface Plasmon Resonance (SPR) biosensor, a thin metal layer (normally a 50- nm-thick layer of gold) is placed on a dielectric material (a crystal), and its functioning is based on the detection of changes in the refractive index around the separation area between both elements.
By exciting the interface between these two layers (under conditions of total internal reflection) a plasmonic resonance[3] is activated with a certain angle of incidence.. This angle, which produces a plasmonic wave (with an evanescent character) is very sensitive to the changes from molecular interactions that happen on the metal surface. Therefore, these interactions between the analyte[4] of the sample and the sensor surface of the biosensor are shown as variations of the resonance angle.
By exciting the interface between these two layers (under conditions of total internal reflection) a plasmonic resonance[3] is activated with a certain angle of incidence.. This angle, which produces a plasmonic wave (with an evanescent character) is very sensitive to the changes from molecular interactions that happen on the metal surface. Therefore, these interactions between the analyte[4] of the sample and the sensor surface of the biosensor are shown as variations of the resonance angle.
Currently, new biosensors are being developed, which rely on the plasmon resonance phenomenon in nanoparticles. While their detection range would be very similar to the SPRs, their functioning system can be made simpler by measuring light transmission instead of light reflection, in addition to the advantage of the device miniaturisation.
Due to the tiny size of nanoparticles, the electron oscillations are more localised than in the previous case (SPR), in specific areas of nanoparticles. This phenomenon is called ‘Localized Surface Plasmon Resonance’ (LSPR). The colour (wavelength: λ) changes that nanoparticles experience by adsorbing[5] the (bio)molecules of the examined sample are used to analyse this sample.
An alternative, within this kind of device,, is DNA sensors detecting colour changes produced by the aggregation of gold nanoparticles marked with stranded DNA complementary to the target DNA.
Nanoplasmonic biosensors are characterised by their real-time detection, specificity, high sensitivity and recognisition rate.
Their main applications are found in veterinarian, biomedical and environmental fields as well as in the food industry.
Their main applications are found in veterinarian, biomedical and environmental fields as well as in the food industry.
Nanomechanical biosensors
These sorts of nanobiosensors use the deflection or the resonance frequency variation of a microcantilever (as a transducing[6] method) when they interact with the studied sample. This position variation (Δx) is just a few nanometers derived from the biomolecular identification of the analysed sample.
Because these microcantilevers can be produced in mass at a low cost by standar microelectronic technology, thousands of them can be manufactured to identify thousands of analytes in a single sample.
The sensor area of these microcantilevers is around 1,000 μm2, which provides them to test sample volume lower than femtomole (10-15 moles).
Both photonic and nanomechanical biosensors could help us to obtain unlimited genetic and proteomic information that would offer the discovery of pathogens, new drugs, vaccines and undiscovered mutations of certain diseases in a faster way than the current technology.
Microfluidic devices or lab-on-a-chip (LOC)
This class of instruments uses an electric field to move liquids, particles, molecules or cells through microcapillaries designed on chips of different materials like silicon, crystal, quartz or plastic.
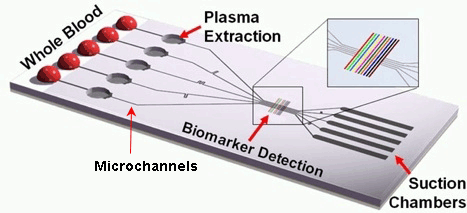
The required volume of the sample fluids is very small, therefore the analysis can be carried out very quickly.
It is a portable and disposable detection method with a high grade of automation.
The ultimate objective for all these biocompatible nanodevices is to finally be implanted in vivo inside our organism, where they would perform a similar function to ‘sentinels’ in the presence of the first diseased cells. Some advances have already been made to that effect at the microscale (pills with built-in image cameras), but, without a doubt, this will become one the major research fields in nanomedicine in the near future.
[1] It describes how light propagates through a certain medium. It is defined as n = c/v, where c is the speed of light in vaccum and v the speed of light in that medium.
[2] Process of programmed cell death.
[3] Excitation or oscillation of conduction electrons at the interface between two materials, in our case a metal layer on a dielectric substrate.
[4] Substance (ion, compound or element) of interest in an analysed sample.
[5] Adhesion of atoms, ions or molecules to a surface.
[6] Process by one signal or energy is converted to another.
[2] Process of programmed cell death.
[3] Excitation or oscillation of conduction electrons at the interface between two materials, in our case a metal layer on a dielectric substrate.
[4] Substance (ion, compound or element) of interest in an analysed sample.
[5] Adhesion of atoms, ions or molecules to a surface.
[6] Process by one signal or energy is converted to another.
Sources: Nanomedicina: aplicación de la nanotecnología en la salud. Laura M. Lechuga. Grupo de Nanobiosensores y Aplicaciones Bioanalíticas
Centro de Investigación en Nanociencia y Nanotecnología (CIN2). CSIC
http://www.kennislink.nl/publicaties/de-opmars-van-de-twentse-lab-on-a-chip
http://images.slideplayer.es/16/5040279/slides/slide_47.jpg
http://www.mdpi.com/sensors/sensors-10-09630/article_deploy/html/images/sensors-10-09630f1-1024.png
https://www.ifm.liu.se/applphys/molphys/research/biosensing_using_nanopart/
http://www.tcd.ie/Physics/people/Martin.Hegner/ReviewNSST-The_impact_of_STM_and_AFM.html?ntherodt_01.pdf
Fritz, J., Baller, M.K., Lang, H.P., Rothuizen, H., Vettiger, P., Meyer, E., Guntherodt, H.-J., Gerber, CH. and Gimzewski, J.K.,
Science 288 (2000) 316.
https://www.theengineer.co.uk/lab-on-chip-device-promises-hiv-diagnosis-in-10-minutes/
Centro de Investigación en Nanociencia y Nanotecnología (CIN2). CSIC
http://www.kennislink.nl/publicaties/de-opmars-van-de-twentse-lab-on-a-chip
http://images.slideplayer.es/16/5040279/slides/slide_47.jpg
http://www.mdpi.com/sensors/sensors-10-09630/article_deploy/html/images/sensors-10-09630f1-1024.png
https://www.ifm.liu.se/applphys/molphys/research/biosensing_using_nanopart/
http://www.tcd.ie/Physics/people/Martin.Hegner/ReviewNSST-The_impact_of_STM_and_AFM.html?ntherodt_01.pdf
Fritz, J., Baller, M.K., Lang, H.P., Rothuizen, H., Vettiger, P., Meyer, E., Guntherodt, H.-J., Gerber, CH. and Gimzewski, J.K.,
Science 288 (2000) 316.
https://www.theengineer.co.uk/lab-on-chip-device-promises-hiv-diagnosis-in-10-minutes/
Your opinion matters